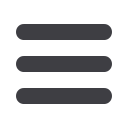
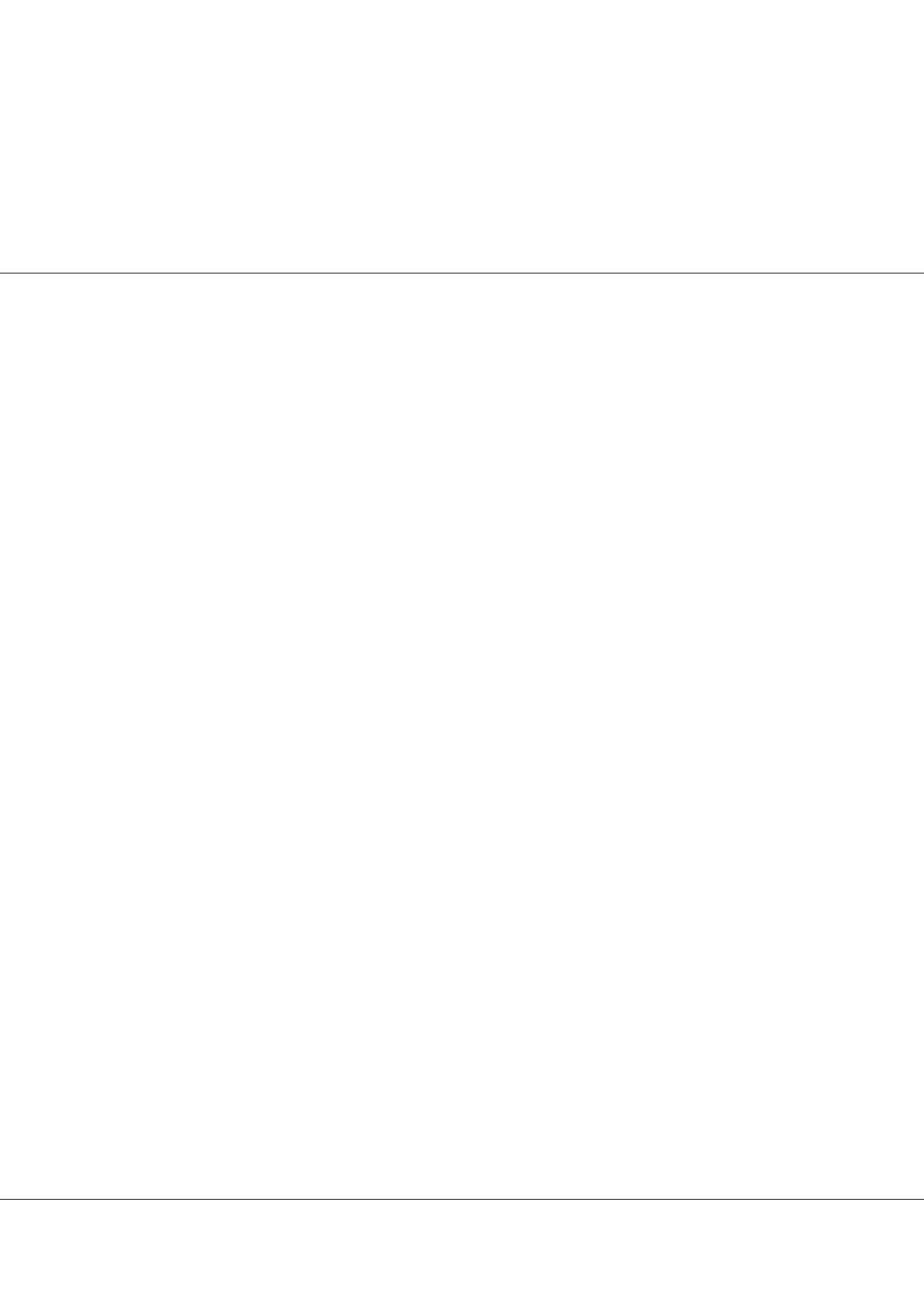
Volume 7, Issue 6(Suppl)
J Chromatogr Sep Tech
ISSN: 2157-7064 JCGST, an open access journal
Page 36
Notes:
Separation Techniques 2016
September 26-28, 2016
conferenceseries
.com
Separation Techniques
September 26-28, 2016 Valencia, Spain
2
nd
International Conference and Expo on
Protonic ceramic membranes under asymmetric steam atmosphere
Sandrine Ricote
1
, Anthony Manerbino
2
and
W Grover Coors
3
1
Colorado School of Mines, USA
2
Solid State Energy, USA
3
Protonetics, USA
A
comprehensive analysis of proton transport in protonic ceramic membrane devices is presented. Thin, dense membranes
of BaZr0.8Ce0.1Y0.1O3-d, BZCY81, may now be fabricated with relative ease at commercial scale. These devices have
potential for supporting the emerging hydrogen economy and reducing dependence of fossil fuels. With protonic ceramic
electrolytes it is possible to galvanically transport pure hydrogen from one side of a membrane to the other, making it
possible to fabricate electrochemical devices and systems that were previously impractical or impossible. H
2
can be produced
from natural gas by steam reforming, whereby hydrogen may be extracted from a reacting stream of methane and steam
in a protonic membrane reformer, PMR; Liquid hydrocarbons, such as ethylene and benzene, may be produced from dry
methane in a catalytic membrane reactor by methane dehydroaromatization, MDA, with hydrogen extracted from the feed
gas; Ammonia can be synthesized by pumping hydrogen through the membrane to react with nitrogen in a process called
solid-state ammonia synthesis, SSAS; and H
2
can be produced from water vapor by steam electrolysis in a protonic ceramic
electrolysis cell (PCEC). In order for these devices to become commercially viable, a clear understanding of their operation
in various use environments is necessary. All the devices listed above consume electric power to pump hydrogen across the
membrane, which must be supplied by an external power source. The power consumed is the product of the applied voltage
and the current consumed by the galvanic device, so it is important that the device have low resistance and high faradaic
efficiency with respect to proton transport and that power is not wasted by parasitic losses. The proton current depends on
the effective resistance, which depends on electrode performance, faradaic efficiency and bulk materials properties. Well-
designed electrodes can, in principle, be developed with low effective resistance, but in the final analysis it is the conductivity
of the electrolyte membrane that limits the performance of these devices. The proton conductivity of BZCY81 is only a few
millisiemens per centimeter in reducing atmosphere. More importantly, conductivity in BZCY is a strong function of water
vapor pressure, making the electrolyte a mixed proton/steam conductor. This is an unusual characteristic that is unique to
protonic ceramic electrolytes. The impact depends on the application. For example, MDA requires nominally dry methane on
the feed side, while PMR requires moist atmosphere with steam-to-carbon ratio greater than unity. SSAS, on the other hand
requires dry atmosphere on the permeate side, where nitrogen reacts with hydrogen to produce anhydrous ammonia. Steam
electrolysis is carried out under moist oxidizing conditions on the feed side. In all cases, the desired permeate is hydrogen, as
dry as possible to avoid the need for separation of hydrogen from steam, but as a practical matter, some steam will be present
either due to steam permeation or added to the sweep gas intentionally. The transport properties of the membrane in each of
these devices depend strongly on pH
2
O and pH
2
on the feed and permeate sides. Knowledge of the electrolyte conductivity as
a function of pH
2
O and pH
2
on each side of the membrane is essential for designing cost-effective galvanic systems since this
determines the protonic flux density.
Biography
Sandrine Ricote has obtained her PhD on Ceramic Proton Conductors at the University of Burgundy, France. She has worked four years in the Department of
Energy Conversion and Storage at the Danish Technical University as a Post-doc and then as a Scientist. In 2012, she moved to the Department of Mechanical
Engineering at Colorado School of Mines. She is currently a Research Associate Professor with a main focus on ceramic proton conductors.
sricote@mines.eduSandrine Ricote et al., J Chromatogr Sep Tech 2016, 7:6(Suppl)
http://dx.doi.org/10.4172/2157-7064.C1.019